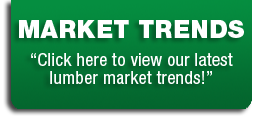
{article.name}
Social Media Links
The Strength of Wood
Wood is exceedingly strong, but it’s a grave mistake to make design decisions based on an assumption that the wood safety factor is excessive.
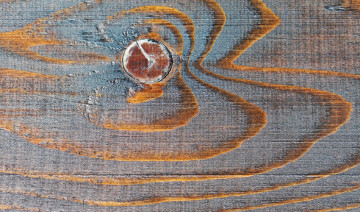
- Share this:
- Share on Facebook
- Pin on Pinterest
- Tweet on Twitter
The strength of wood is typically measured by a number of "strength properties," including:
- Modulus of rupture in bending - This reflects the maximum load-carrying capacity of a wood framing member in bending, and is proportional to maximum moment borne by the test specimen.
- Work to maximum load in bending - Ability to absorb shock with some permanent deformation and more or less injury to a specimen. Work to maximum load is a measure of the combined strength and toughness of wood under bending stresses.
- Compressive strength parallel to the grain - Also know as the maximum crushing strength, this is the maximum stress sustained by a compression parallel-to-grain specimen having a ratio of length to least dimension of less than 11.
- Compressive stress perpendicular to grain - Maximum stress sustained by a compression perpendicular-to-grain. This gets reported as a proportional value. There is no clearly defined ultimate stress for this property.
- Shear strength parallel to grain - The ability to resist internal slipping of one part upon another along the grain. Values presented in design tables are average strength in radial and tangential shear planes.
- Tensile strength perpendicular to grain - Resistance of wood to forces acting across the grain that tend to split a member. Values presented are the average of radial and tangential observations.
- Tensile strength parallel to grain - Maximum tensile stress sustained in a direction parallel to grain. Relatively few data are available on the tensile strength of various species of clear wood parallel to grain. In the absence of sufficient tension test data, modulus of rupture values are sometimes substituted for tensile strength of small, clear, straight-grained pieces of wood.
Like so many properties of wood, the basis for these measurements assumes clear, straight-grained wood. But because of the natural growth characteristics of trees, wood products used for building structures vary due to knots and the localized slope of grain, plus a number of natural defects caused by biological or climatic elements influencing the living tree.
Grading Lumber
Lumber producers have graders who grade every piece of lumber as it is produced. The key factors considered in the grading process are knots and slope of grain. Grading also considers a low number of growth rings per inch in some species (for example, southern pine) as a visual indication of the presence of low-density material and, to some extent, juvenile wood. The regional inspection groups, which monitor the process, visit each mill at least 10 times a year to check the grading. These regional groups contend that today’s mill-based inspectors are better trained and more sophisticated now than at any time in the past. As a result of this improved inspection process, structural lumber reaching the lumber yards today is more “on grade” than in years past. So even if some of today’s lumber looks worse than in days gone by, builders have great assurance that a piece of lumber graded “No. 1” will perform at its grade level.
Knots and the slope of grain are two of the characteristics used to visually sort lumber into stress grades. Other characteristics that go into the stress grading of a piece of lumber include splits that appear as the lumber dries, shake, density, decay, annual ring count and percentage latewood, pitch pockets, and wane.
Knots
The influence of a knot on the mechanical properties of a wood member is due to the interruption of continuity and change in the direction of wood fibers associated with the knot.

The influence of knots depends on their size, location, shape, and soundness; attendant local slope of grain; and type of stress to which the wood member is subjected. The shape (form) of a knot on a sawn surface depends upon the direction of the exposing cut. A nearly round knot is produced when lumber is sawn from a log and a branch is sawn through at right angles to its length (as in a flatsawn board). An oval knot is produced if the saw cut is diagonal to the branch length (as in a bastard-sawn board) and a “spiked” knot when the cut is lengthwise to the branch (as in a quartersawn board).
Although a knot itself is as strong as the rest of a piece of wood, the area near the knot is weak because of crossgrain. If a piece of lumber is milled when the log is still green, the knot is said to be “tight.” If the growth layer of the tree is dead, however, the knot will be loose or fall out when sawn.
In general, knots have a greater effect on strength in tension than compression; in bending, the effect depends on whether a knot is in the tension or compression side of a beam (knots along the centerline have little or no effect).

Grading rules limit the number of knots in structural lumber because the distortion of grain around the knot reduces a board’s strength. All these knots are in #2 SPF: A tight, intergrown knots (left); a spike knot (center); and an encased knot (right). The encased know will resist (or transmit) little or no stress, but there is usually less distortion of grain around one, and therefore it is graded as equivalent to a tight knot of equivalent size.
Intergrown (or live) knots resist (or transmit) some kinds of stress, but encased knots (unless very tight) or knotholes resist (or transmit) little or no stress. On the other hand, distortion of grain is greater around an intergrown knot than around an encased (or dead) knot of equivalent size. As a result, overall strength effects are roughly equalized, and often no distinction is made in stress grading between intergrown knots, dead knots, and knotholes.
In tension, knots along the edge of a member cause an eccentricity that induces bending stresses, and they should therefore be more restricted than knots away from the edge. In simply supported structural members subjected to bending, stresses are greater in the middle of the length and at the top and bottom edges than at midheight. These facts are recognized in some grades by differing limitations on the sizes of knots in different locations.

Laminated veneer lumber (LVL), like the structural beam being installed here, is stronger than comparably sized sawn lumber because any natural defects like knots and splits are only one ply deep.
Knots have a much greater effect on strength in axial tension than in axial short-column compression, and the effects on bending are somewhat less than those in axial tension. For this reason, in a simply supported beam, a knot on the lower side (subjected to tensile stresses) has a greater effect on the load the beam will support than does a knot on the upper side (subjected to compressive stresses). In long columns, knots are important because they affect stiffness. In short or intermediate columns, the reduction in strength caused by knots is approximately proportional to their size; however, large knots have a somewhat greater relative effect than do small knots. Knots in round timbers, such as poles and piles, have less effect on strength than do knots in sawn timbers. Although the grain is irregular around knots in both forms of timber, the angle of the grain to the surface is smaller in naturally round timber than in sawn timber. Furthermore, in round timbers there is no discontinuity in wood fibers, which results from sawing through the grain.
Slope of Grain
The term slope of grain relates the fiber direction of the wood relative to the edge of a piece of wood. In ordinary lumber, two important forms of cross grain are spiral and diagonal, though there are other forms, such as wavy, dipped, interlocked, and curly.
Slope of grain reduces the mechanical properties of lumber because the fibers are not parallel to the edges. Severely cross-grained pieces are also undesirable because they tend to warp with changes in moisture content. Stresses caused by shrinkage during drying are greater in structural lumber than in small, clear straight-grained specimens and are increased in zones of sloping or distorted grain. To provide a margin of safety, the reduction in design properties resulting from cross grain in visually graded structural lumber is considerably greater than that observed in small, clear specimens that contain similar cross grain.
Spiral grain is caused by winding or spiral growth of wood fibers about the bole of the tree instead of vertical growth. In a sawn board, spiral grain can be defined as fibers lying in the tangential plane of the growth rings, rather than parallel to the longitudinal axis of the board. A visual method of determining the presence of spiral grain is to note the alignment of pores, rays, and resin ducts on a flatsawn face. Drying checks on a flatsawn surface follow the fibers and indicate the slope of the fiber.
Diagonal grain is cross grain caused by growth rings that are not parallel to one or both surfaces of the sawn piece. Diagonal grain is produced by sawing a log with pronounced taper parallel to the axis (pith) of the tree. Diagonal grain also occurs in lumber sawn from crooked logs or logs with butt swell.
Interlocked grain. A regular reversal of right and left spiraling of grain in a tree stem produces the condition known as interlocked grain. Interlocked grain occurs in some hardwood species and markedly increases resistance to splitting in the radial plane. Interlocked grain decreases both the static bending strength and stiffness of clear wood specimens. The presence of interlocked grain alters the relationship between bending strength and compressive strength of lumber cut from tropical hardwoods.
Other common defects due to the natural growth of trees include:
Bark pockets are small patches of bark embedded in dressed lumber. They usually affect only the appearance of the piece, but they can reduce the withdrawal strength of a nail or screw. Grading rules limit the amount of bark, but because of its location near the outside of the tree, it may pass graders’ tests for strength and stiffness.

Bark pockets often occur when bark gets trapped by the tree’s growth at the crotch of a tree, evident here by the grain pattern of the center board.
Checks and splits. Checks are separations of the wood that normally occur across or through the annual rings, usually as a result of seasoning. Splits are a separation of the wood through the piece to the opposite surface or to an adjoining surface caused by tearing apart of the wood cells. As opposed to shakes, checks and splits are rated by only the area of actual opening. An end-split is considered equal to an end-check that extends through the full thickness of the piece. The effects of checks and splits on strength and the principles of their limitation are the same as those for shake.
Shake is a separation or a weakness of fiber bond, between or through the annual rings, that is presumed to extend lengthwise without limit. Because shake reduces resistance to shear in members subjected to bending, grading rules therefore restrict shake most closely in those parts of a bending member where shear stresses are highest. In members with limited cross grain, which are subjected only to tension or compression, shake does not affect strength greatly. Shake may be limited in a grade because of appearance and because it permits entrance of moisture, which results in decay.
Density. Strength is related to the mass per unit volume (density) of clear wood. Properties assigned to lumber are sometimes modified by using the rate of growth and percentage of latewood as measures of density. Typically, selection for density requires that the rings per unit length on the cross section and the percentage of latewood be within a specified range. Some very low-strength pieces may be excluded from a grade by excluding those that are exceptionally low in density.
Decay in most forms should be prohibited or severely restricted in stress grades because the extent of decay is difficult to determine and its effect on strength is often greater than visual observation would indicate. Some decay that occurs in knots may be permitted if the decay does not extend into the surrounding wood.
Heartwood and sapwood. Heartwood does not need to be taken into account in stress grading because heartwood and sapwood have been assumed to have equal mechanical properties. However, heartwood is sometimes specified in a visual grade because the heartwood of some species is more resistant to decay than is the sapwood; heartwood may be required if untreated wood will be exposed to a decay hazard. On the other hand, sapwood takes preservative treatment more readily than heartwood and it is preferable for lumber that will be treated with preservatives.
Pitch pockets ordinarily have so little effect on structural lumber that they can be disregarded in stress grading if they are small and limited in number. The presence of a large number of pitch pockets, however, may indicate shake or weakness of bond between annual rings.
Wane refers to bark or lack of wood on the edge or corner of a piece of lumber, regardless of cause (except manufactured eased edges). Requirements of appearance, or the need for full edge bearing generally impose stricter limitations on wane than does strength. Wane is therefore limited in structural lumber.

Wane, shown her on a ceiling joist, is common on ungraded framing stock in old buildings. However, it is rarely a structural problem except when it reduces the bearing area of a beam.
Overcoming Defects
In the design of a wood structure, engineers apply safety factors to account for the reduced strength. Since you can't test and tabulate every defect in the lumber used in a building, here's how safety factors work:
To arrive at the design values used in wood building design, thousands of pieces of lumber of representative sizes, grades, and species were tested. These tests are run for about 10 minutes to determine the stress that will cause a piece of lumber to fail. The test data for every piece of lumber of a given grade, size, and species is recorded. In a test of bending strength, for example, the values from a batch of lumber might range from 3,000 to 15,000 psi. By convention, the value of the 5th percentile is calculated (in other words, 95% of the pieces tested fall above this number, 5% fall below). Choosing a value at the 5th percentile is a way of accounting for the wide variability in the strength of pieces of visually graded lumber (due to knots, slope of grain, etc.).
This number — let’s say it’s 4,000 psi — is then divided by 1.62 to convert it to a 10-year duration value, which is the load duration that is used in the design of wood floor systems. (Remember, the test lasts only 10 minutes; lumber can resist more stress for short periods of time.) Finally, the 10-year value is divided by a safety factor of 1.3. So a 5th percentile value of 4,000 psi would become 1,899 psi. This is the number that is published in the allowable design stress tables.
It’s a grave mistake to make design decisions based on an assumption that the wood safety factor is excessive.
Sign up for our Email List
Stay updated with all our latest posts, products and offers! Just enter your information below.
Comments